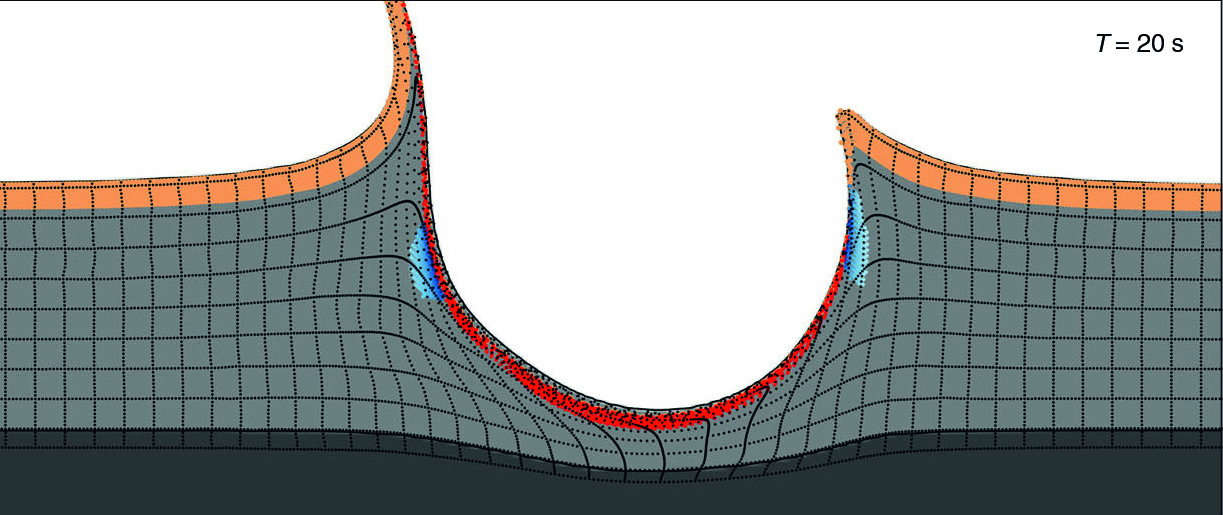
Collins et al./Nature Communications
Tracing the trajectory of a 66 million-year-old asteroid impact
Unsurprisingly, the reconstructed angle was a worst-case scenario.
by Scott K. JohnsonYou know that scene in every forensic crime drama where someone works out the angle the bullet was fired from and points back to the source? In the case of the Chicxulub asteroid impact and the end-Cretaceous mass extinction 66 million years ago, there’s no mystery about the shooter. (Space did it.) But the trajectory is interesting for other reasons, and researchers have long been trying to trace the path back out of the crater off the Yucatán coast.
Unsurprisingly, 66 million years have taken their toll on the crater, so researchers have offered several very different answers. Did the asteroid hit from the southeast at a very low angle? Did it come from the southwest at a moderate angle? Many studies that needed to model the impact have simply defaulted to a 90-degree strike and avoided the whole argument. The details actually matter, though, and precisely which rocks get vaporized—and which climate-changing gases they release—depends on that impact angle.
A lot of new research on the crater has been published recently thanks to a major expedition that included drilling a rock core down through the crater’s peak ring. (Impacts this violent leave a raised ring in the center rather than a single peak.) A new paper led by Imperial College London’s Gareth Collins makes the latest contribution using model simulations to see what impact angle best matches the observed characteristics of the crater.
Out of alignment
To uncover the geometry of what is left of the crater, which is partly on land and partly on the seafloor, scientists turn to sonar-like seismic imaging and extremely precise gravity measurements. Seismic imaging highlights features like layers of sediment and rock, as well as faults. Variations in gravity tell scientists about the density of the rocks beneath them. In this case, that happens to include pin-pointing the location where the Earth’s mantle rises closest to the surface.
All this allows three critical points to be plotted on the map: the geometric center from the crater rim, the center of the peak ring, and the high point of the mantle. In a perfectly 90-degree strike, these three points would overlap. The peak ring would form a dead-center bullseye in the crater, and the mantle high spot would be right beneath it—the product of a gelatin-like vertical rebound after the tremendous force of the impact.
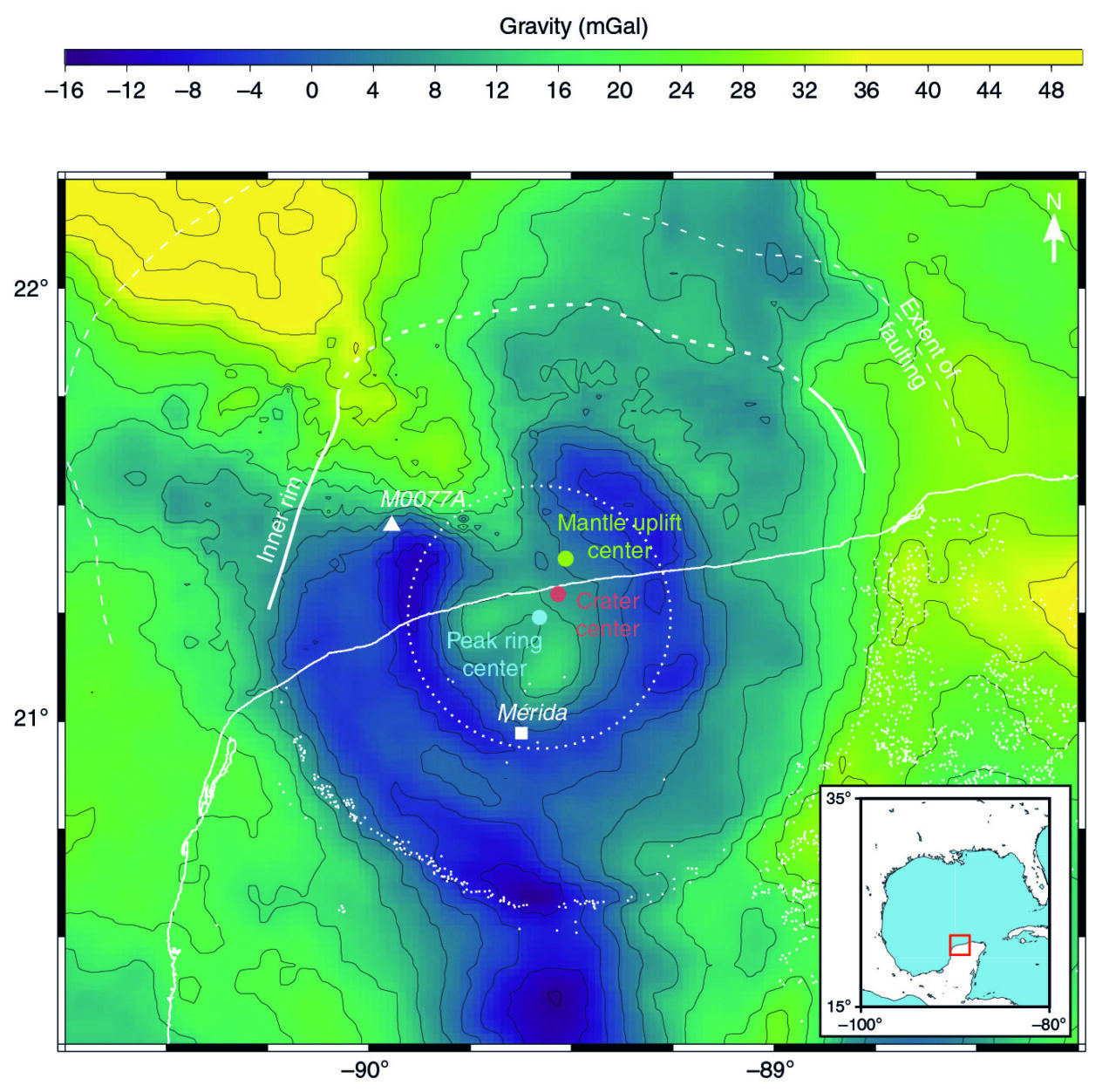
Collins et al./Nature Communications
Since they aren’t lined up, the question is what combination of impact angle and direction puts the peak ring center just southwest of the crater center, with the mantle rebound high point just northeast of the crater center. To test this, the researchers used a 3D simulation of the extreme physics of an asteroid impact. They repeated the simulation for impacts at 90-, 60-, 45-, and 30-degree angles and at velocities of 12 kilometers per second (the minimum plausible) and 20 kilometers per second.
Within just a few minutes, the impact creates a “transient crater” some 20 kilometers (12 miles) deep while throwing up a temporary mountain range, after which things bounce back to form a shallower final crater.
Impacts at 60 and 45 degrees result in the mantle high point and the peak ring center being offset on opposing sides of the crater center—just like we see in the real crater. But at 30 degrees, the mantle high point actually joins the peak ring center over on the other side, so they are both offset in the same direction.
Angled impact
There’s also a visible difference in the peak ring itself. The 60- and 45-degree impacts show some of the outer sedimentary rocks near the surface getting “sucked” down beneath deep rocks pushing up from the center. Outside the crater, sedimentary rocks lie on top of deeper granites, but this relationship is flipped in the peak ring. That’s exactly what the rock core from the recent expedition showed. But in the 30-degree impact simulation, the deep-on-top pattern doesn’t materialize on the downrange side of the crater.
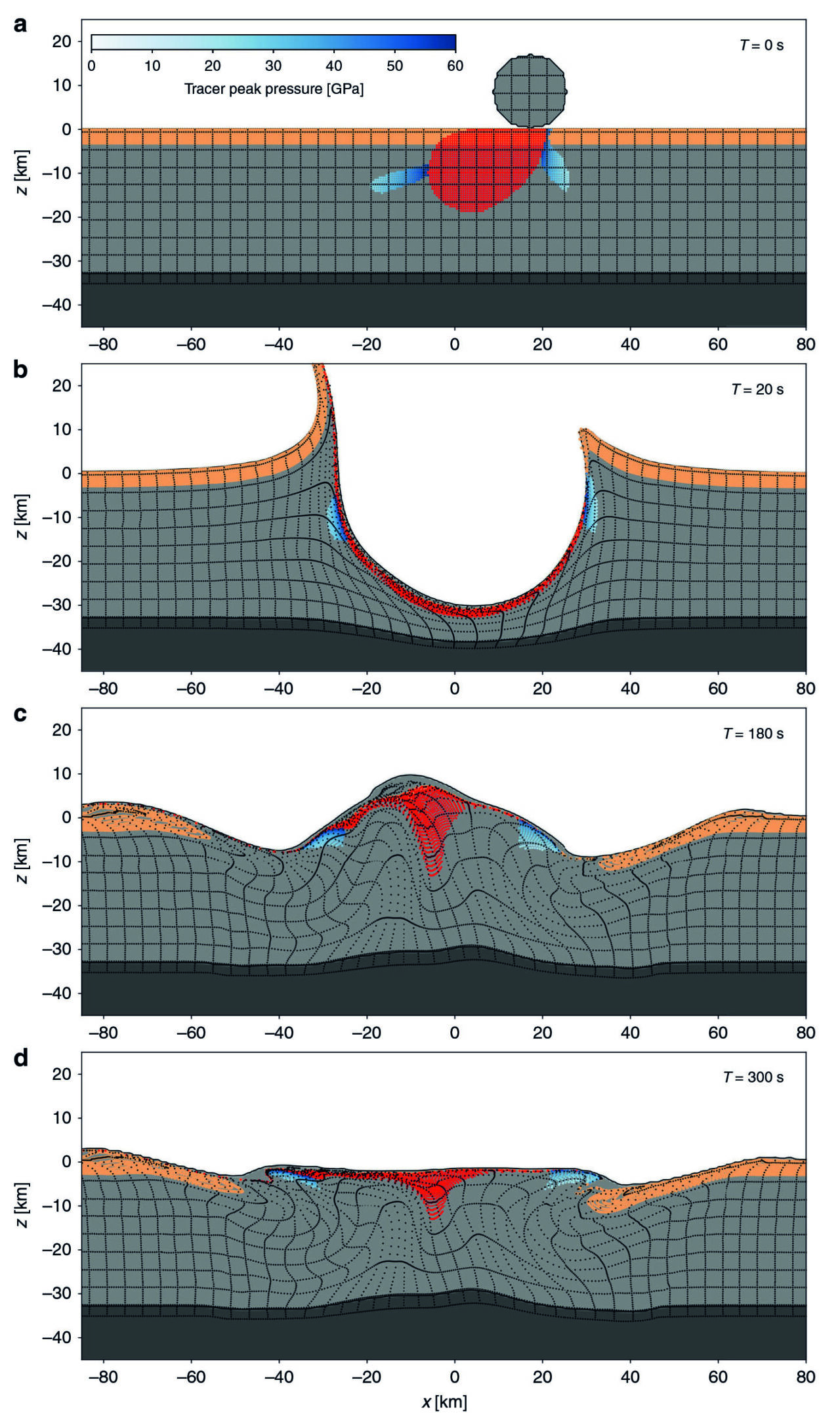
Collins et al./Nature Communications
Put together, these results point to an impact angle closer to 60 degrees, ruling out some earlier ideas about a low-angle strike. But the simulations also challenge conclusions that the impact came from the southwest. They show that the mantle high point marks the uprange direction and that the peak ring center marks the downrange side. In the case of the Chicxulub crater, that means the impact came from the northeast.
As it happens, this intermediate impact angle would maximize the climate impact of the event. Given the depths of the relevant rock layers that were vaporized, this impact would release about 2 to 3 times as much sulfur (causing short-term cooling) and CO2 (causing long-term warming) as a 90-degree strike. And compared to a super-low-angle 15-degree impact, it would release about 10 times more. Looking at how the mass extinction event played out, it would seem that release was sufficiently effective.
Nature Communications, 2020. DOI: 10.1038/s41467-020-15269-x (About DOIs).